I have another post about cathodes with some analogies about coffee filters and jenga blocks, that is very non-technical. Please read that first if you don't know anything about batteries. If it's interesting to you, here I want to go a different approach and explain a the type of cathode I know most about (NMC) and get pretty technical with it.
But first a bit about the other cathode, not just NMC. There are lots of cathodes for all sorts of different applications, but generally speaking when it comes to electrical vehicles, there are two main types of Li-ion battery cathodes, LFP and NMC.
LFP
Lithium Iron (Fe) Phosphate
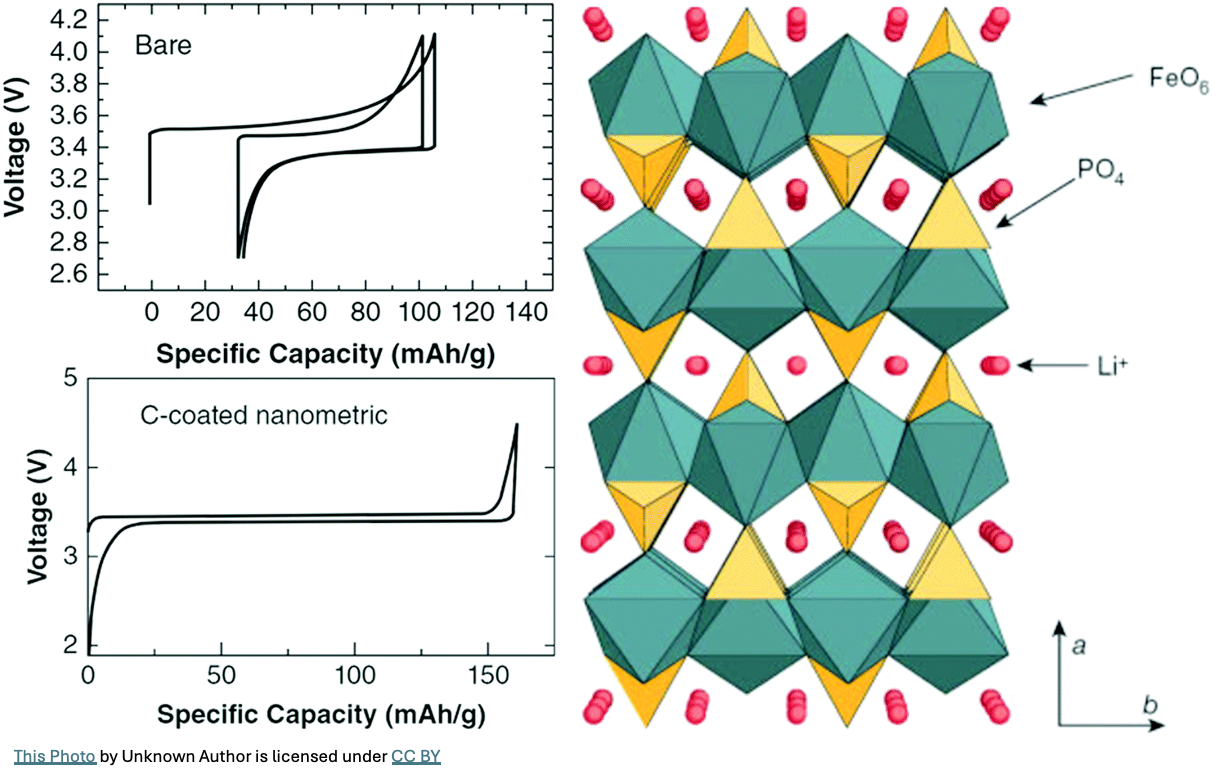
LFP has been around for a while and as the price of lithium has dropped from new mining endeavors, it has become very cheap and many EV manufacturers, including Tesla, have chosen to use it in their budget-tier cars.
LFP consists of lithium (L), iron (F), and phosphate (P), all of which are relatively cheap. Generally speaking, LFP is safer, cheaper, more environmentally friendly, and lasts longer (more charges) than NMC, but it misses the mark compared to NMC on two of the most important factors, power and energy which translate to horsepower and range in the context of electric vehicles.
For that reason, LFP works for a lot of cases if people are comfortable with low range, low horse power applications. But for lots of other electric applications, planes, trucks, long range vehicles etc, LFP as it is now, might not cut it. There's lots of interesting stuff going on to make LFP better in these regards, but it's not my expertise so I won't get into it.
NMC
Nickel Manganese Cobalt
NMC has in some form existed since the 80s and basically ushered in the portable electronics revolution, which would have been impossible without it, though back then it only contained lithium and cobalt. Even now, most smartphones and laptops, including iPhones and Macbooks work on this effectively unchanged NMC cathode from 90s, LiCoO2
For portable electronics, this works, because the devices are small and don't require huge batteries, but for electric vehicles, which require orders of magnitude larger batteries than phones, cobalt could never be the solution.
Over the years, academia and industry have realized that adding nickel (N) and manganese (M) to replace some portion of the cobalt (C) in these cathodes makes them a lot cheaper. It's also more environmentally friendly and ethical while preserving the cathodes' most desirable traits. In fact, some modern cathodes have practically none or very little cobalt at all. Nickel generally controls the price point of these cathodes now as it is the next most expensive raw material in them after cobalt.
Most NMC cathodes provide longer range and higher power than LFP and so they are considered premium, but not all of them are equal. The more nickel there is, there is generally high capacity (range), and the more manganese, there is generally high power (horsepower).
Most of the German auto manufacturers (except maybe VW) which are considered premium as well as other manufacturers for their high-end models will most likely use NMC.
Okay now the fun part!
Structure
NMC works well for a lot of reasons as a cathode, but one of its signature traits is its layered structure. LFP has a complex structure, and it works fine actually, but NMC is much simpler. It's effectively like an enormous bookshelf.
As we'll see later, this makes it very easy for lithium ions to move around, but first some technical background on oxidation.
Oxidation
Oxidation state is one of the most important aspects of cathodes. It's a complicated concept, and distinctly different from the real "charge" of an ion (charged atom). What oxidation state basically represents is an ion's (charged atom) loss/gain of electrons. A positive number indicates loss of electrons and a negative number indicates an abundance. Oxidation states are inferred and more something the ion experiences than a trait that it has itself. There are also default oxidation states, which I will go over below. These are not entirely accurate and absolutely an oversimplification, but good enough for our purposes.
The oxidation numbers should balance to 0 across the whole cathode, meaning that if electrons leave or enter the cathode, they must be coming or going into somewhere and changing some ion's oxidation state. For instance, a common NMC cathode is NMC 622. In this cathode, for every 100 lithium, there is 60 nickel, 20 manganese, 20 cobalt, and 200 oxygen, leaving the formula as this:
Li1.0Ni0.6Mn0.2Co0.2O2.0
In this NMC 622, the oxidation states look something like this:
Element | Oxidation State |
---|---|
Lithium | +1 |
Nickel | +2 (+2.4) |
Cobalt | +3 |
Manganese | +4 |
Oxygen | -2 |
This is all theoretical; in reality nickel in particular probably has an average oxidation state around +2.4 meaning that some nickel ions have an oxidation state of +3 and not +2 (fractional oxidation state can't exist in reality).
Given these approximations, the overall balance of the cathode is 0. This calculation is simple using the amount of each ion and its oxidation state above:
(1)(1) + (2.4)(0.6) + (4)(0.2) + (3)(0.2) + (-2)(2) = 0
Mostly, in the case of NMC, changes in oxidation happens through nickel and cobalt, and so electrons that leave or come in to the cathode generally do so through these two ions. Sometimes it can also occur in oxygen or manganese at very high voltages. But focus on nickel for now. Let's go through an example.
Charging the cathode
In the base cathode, just put into a battery, full of lithium, fresh from firing, we consider that the oxidation state of every nickel ion is +2.
The first step is to charge the battery, which means chemically pulling lithium ions out of the cathode and inserting them into the anode. In practice, this means plugging the battery into an outlet which will use an external voltage to force electrons out of the cathode and into the anode.
Lithium ions will begin to leave the cathode. This is because they are much much smaller than the other ions and within the structure exist in huge hallways where they can leave the cell in a very orderly fashion indeed. In fact, new research even shows that when the lithium ions leave the cell, those hallways get even bigger as the oxygen layers repulse one another more and more since there is no lithium in between them.
So the lithium ions are moving through the electrolyte and over to place themselves into the anode. Once they leave, however, there must be balance in oxidation state and so one of the other elements must change its oxidation state. Of the mostly nickel, manganese, and oxygen (let's ignore in the cathode, nickel is the easiest to change from +2 to +3 and so that transformation begins. By the time around 1/3 of the original lithium has left the cathode, almost all of the nickel has changed to oxidation state +3.
What happens next as more lithium leaves is not entirely clear, but at least initially, it is still easier for nickel to change again, from +3 to +4 than for manganese to switch to +5 or oxygen to come to -1. So nickel continues changing until around half of the original lithium is gone. At that point nickel continues to go to +4 but there will also be changes in the oxidation state in cobalt occurring as well as it goes from +3 to +4. As more and more lithium goes, some academic papers have observed that manganese and oxygen will start to change as well with oxygen in particular going from -2 to -1 in some cases.
Where to stop "charging" the Li-ion battery is important. In reality, you can't pull all of the lithium out. First and foremost, to return to the Jenga analogy, this would certainly cause a collapse of the structure of the cathode.
In reality, as more and more lithium is pulled out, the cathode begins "resisting" more and more. This occurs because it becomes more and more difficult for the remaining ions to account for the change in oxidation state - for every additional lithium ion pulled out, you now need to convince an ion like manganese, oxygen, or a very high oxidation state cobalt or nickel to go to an even higher oxidation state and they are not wild about this. In practice, if you do force these ions to even higher oxidation states, the cathode will begin to change shape and collapse which would mean that you could maybe use it one time and then it would not be chargeable again. In reality, this change of cathode shape transformation that happens over time, is generally what causes batteries to need to be replaced.
Also at these high voltages, the other portions of the battery start to have reactions to make up for the lack of charge balance, particularly the electrolyte. This is no good, and can lead to overheating or just generally bad battery life.
For this reason, most commercial cathodes on charge are meant to take much less than 100% of the lithium out of the cathode. The number depends but is maybe closer to 70%, leaving 30% of the lithium still in the cathode even at full charge.
Discharge
On discharge, this whole process happens in reverse; the difference is only that now there need not be any external source. The cathode would like to get those electrons back and take the lithium back to go to its resting state, but until the cathode and anode are connected with a copper wire, the electrons can't flow and thus that transformation can't occur.
Once a device is connected to the battery, however, that process can begin, sending electrons through the device, let's say a phone, and the lithium ions moving back from anode to cathode, into their even large hallways in the cathode. This process will continue until all of the nickel and cobalt have gone from +4 back to +2 and +3 respectively.
Again, this process likely won't go to completion, the phone may have software or a small resistance to make sure that not all of the lithium is forced back into the cathode, for similar structural reasons as mentioned above, but in this case, it will be close, something around 95%.
So all in all around 65% (95%-30%) of the lithium moves back and forth between cathode and anode during one charge-discharge cycle.
Element Roles
What does each element do?
In the NMC cathodes, every element has a role, it looks something like this:
Element |
Role | Why? |
---|---|---|
Lithium | Charge carrier | Small and light and not crucial to structure |
Nickel | Charge balancer | Easy to change charge while maintaining structure |
Manganese | Structure stabilizer | Not willing to change and stays strong for the rest of the structure |
Cobalt | Charge balancer | Easy to change charge while maintaining structure |
Oxygen | Negative balancer | Balances the structure with consistent negative charge and maintains structure |
Nickel and cobalt act as charge balancers that can adjust as the charge carrier, lithium, moves throughout charging and discharging.
Manganese is mostly considered a structure stabilizer; because of its size and high oxidation state, it forms the layered structure with these other compounds. It is generally used as opposed to other structural aids because it is very cheap.
Oxygen is considered an "anion" as opposed to the rest of the "cations" due to its negative charge. For this structure to form at all, some anion must be present, and oxygen is cheap and easy to work with. Increasingly literature indicates that oxygen plays a profound and unexpected role in NMC batteries, participating in the same "redox" (reduction and oxidation which means gaining or losing electrons i.e changes in oxidation state) reactions as nickel and cobalt, only in this case it happens at high voltages when most lithium has left the cell.
There is a whole periodic table of elements to try in these structures, and the combinations are effectively endless. This is exactly what researchers do; in industry, there's no reason to try the expensive elements like gold or platinum as they could never be cost competitive, but academics will try just about anything to see if there's a change in result and then they have to ascertain "why."
In some cases, these elements are "doped" into the cathode at very small levels as in maybe just 1 for every 1000 lithium ions (believe it or not this can have a big effect). Other times, in the case of iron for example, it is added in as a replacement to nickel or cobalt at an amount as high as 10 iron ions per 100 lithium ions.
Titanium for example is often used as another stabilizer ion as it has similar traits to manganese.
Increasingly, manufacturers have a secret sauce in their cathodes. It's already non-trivial to reverse engineer a cathode to see what the ratio is of the elements inside, but it's even harder to figure out the processing conditions that were used to make it like that.
Density
For every lithium ion that moves during discharge, one electron can travel through the circuit. So if as in the above example we can shuttle 65% of the total lithium back and forth sustainably, then we get that many electrons, and thus that much electricity. There's no two ways about it!
We want to get this electricity number as high as possible for a given cathode, because we are always also trying to optimize for density. Cathodes are heavy (they are full of metals after all) and they can also be quite large in volume. Ideally, we want the smallest possible cathode and the lightest possible cathode that can deliver the highest possible power and energy. Remember, power is how fast the car can accelerate, and energy is how far the car can go.
We also want the cathode to cost as little as possible/per the amount of energy and power we get.
This leaves us with six different densities:
Property | Metric | Density |
---|---|---|
Weight | Energy | Gravimetric energy density |
Weight | Power | Gravimetric power density |
Size | Energy | Volumetric energy density |
Size | Power | Volumetric power density |
Cost | Energy | Price energy density |
Cost | Power | Price power density |
These densities are always optimized for any given cathode, and which is important depends on the application.
For example, for phones, cost of the battery is small compared to all the electronics since the battery itself only needs to power a small device for about a day, so it's fine to use all cobalt batteries because they can make the battery both as light as possible and small as possible for still very good metrics.
For cars on the other hand, price matters a lot, since cars need much larger batteries, but weight matters very little and size is not as important, hence why LFP (much larger size than NMC) is suitable for most cheap car alternatives, but there is still some balance needed, for more premium cars, NMC622 or even 811 could be better choices.
Finally, in the case of stationary storage, that is, batteries to store energy from solar, wind etc, size and weight matter practically not at all since these are usually huge facilities in the middle of nowhere, and so generally the cheapest batteries possible are used. In this case even the lithium in LFP is too expensive so it looks like mainly sodium batteries will take this market.